We were taught in school that there are three states of matter: solid, liquid, and gas. Upon heating, a material in the solid state can undergo two phase transitions. First, it can melt when going from solid to liquid, and then it can evaporate when going from liquid to gas. However, when one goes beyond this (very useful) simplification, one learns that additional states of matter exist, e.g. plasma and Bose-Einstein condensate. For those of us interested in the material sciences, the picture begins to get even richer. First, we learn that solids can be divided into two categories: crystalline solids (ordered in the atomic/molecular level, e.g. table salt, ice crystals or diamonds) and amorphous solids (disordered/random, e.g. glass or plastics). Furthermore, we learn that for certain materials, an intermediate phase between the crystalline solid and the liquid also exists. It is called the liquid crystalline phase. Then we learn that this state is not unique, that a whole hierarchy of liquid crystalline phases exist, the simplest of which is the so-called nematic phase. And finally we learn that, despite being widely theoretically predicted and searched for, a "simple" polar nematic phase has never been experimentally found. Until now, that is.
A Bit of Background
As with any good story, research on liquid crystals (LCs) started by accident. In 1888, when studying the function of cholesterol in plants, the Austrian botanists Friedrich Reinitzer observed that an organic cholesterol derivative (cholesteryl benzoate), when heated, had two melting points. First, it melted from the crystalline state into a cloudy liquid and then, at higher temperature, into a clear liquid. Intrigued by this behaviour, Reinitzer wrote a letter to the German physicist Otto Lehmann, who had constructed a heating stage for his polarizing optical microscope a few years earlier, an important technological development at the time. Lehmann studied Renitzer's materials with his polarizing microscope and observed that the intermediate cloudy liquid, while still flowing, also manifested the optical properties of crystals (more on this below). This intermediate condition led him to label such substances as "flüssige Kristalle", which are today widely known as Liquid Crystals.
Interest in this kind of materials started to grow thanks to the contribution of Daniel Vorlander, leader of a group of chemists in the Martin Luther University in Halle, who had been synthesizing many new liquid crystalline materials and identifying what molecular features give rise to these phases. Experimental work on physical properties of liquid crystalline materials was then supported by the theoretical work of Emil Bose, Max Born, Carl Oseen, and F. Charles Frank among others. In the first half of the nineteenth century, great progress was made explaining their structure and degree of order, their behaviour under electric and magnetic fields, their light scattering and elastic properties. As Frank himself noted, interest in liquid crystals diminished after the end of WWII, with many scientist believing that everything about them had been solved and they remained little more than a scientific curiosity.
That changed in the 1960s. In addition to basic research, RCA Laboratories in Princeton presented the first prototype of a liquid crystal display (LCD). The main problem with this first LCD was that it had a working temperature of 125°C, because LC materials at the time only showed suitable LC phase at high temperatures. In subsequent years, chemists' efforts led to the successful design and synthesis of materials with a stable nematic phase at room temperature (molecules as MBBA by Hans Kelker in 1969 and the nCBs by George Gray in 1973), and this opened the door to commercial LC display technologies. Suddenly something that had been a mere curiosity, entered the life of nearly everyone on the planet in the form of LCDs in watches (who did not own a Casio!), calculators, flat televisions, phones, etc.
So: What are Liquid Crystals?
The main difference between crystals and liquids is related to how the molecules are arranged in these states. In a crystalline solid, the molecules have positional and orientational order: i.e. they occupy specific sites in a 3D lattice and their molecular axes point in specific directions. Conversely, in liquids, molecules can diffuse freely within the sample container (no positional order) and the molecules do not show any orientational correlation with respect to other molecules (isotropic liquid). Interestingly, many intermediate phases exist in nature with less order than crystals and more than liquids. These phases are called LC phases. In LC phases, molecules or groups of molecules flow as they do in liquids, while a certain degree of orientational and even long-range positional order is present as in solids. LC order is relatively small when compared to crystals but significant enough to result in fascinating properties when compared to isotropic liquids.
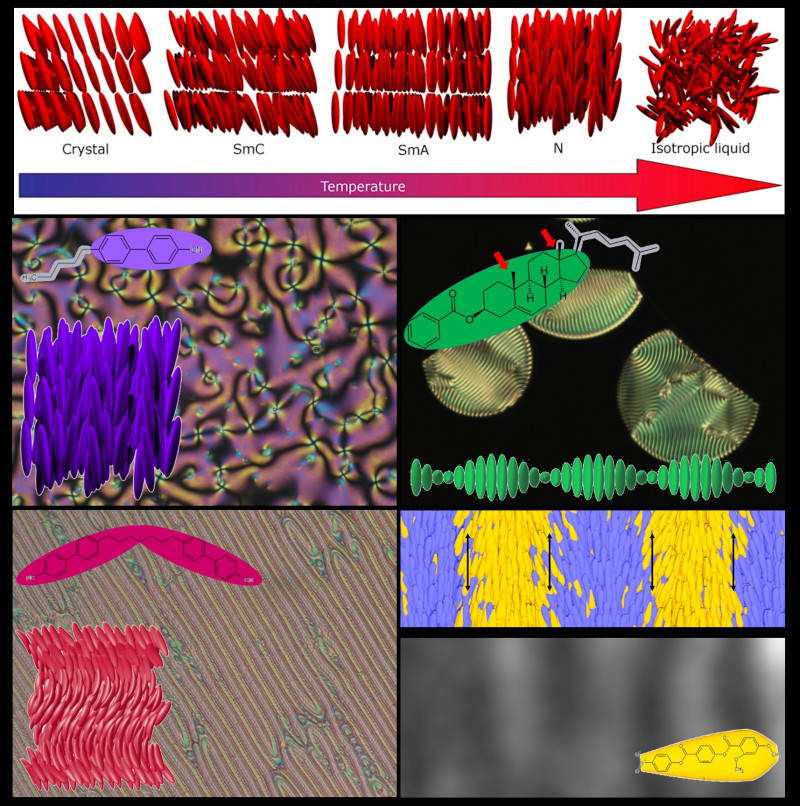
LC phases are classified depending on the orientational and positional order of the molecules. The simplest and least ordered of the liquid crystal phases is the nematic phase (N), where the molecules orient on average along a preferred direction and their positions are randomly distributed and free to diffuse. While the molecules all orient on average in the same direction, their electrical dipole moments can be pointed either in the same direction or in the opposite direction. The nematic phase therefore possesses so-called tail-to-head symmetry and consequently any spontaneous polarization is cancelled out, preventing the appearance of polar phases. The next of the liquid crystalline phases are smectic phases (Sm) or lamellar phases. In addition to the orientational order, smectic phases retain some degree of positional order while remaining fluid, i.e. the centre of mass of the molecules arranges itself in layers while still moving freely within them. Molecules can orient themselves within the layers with their axis perpendicular to the layer plane (SmA) or tilted (SmC). This tilt can be the same from layer to layer, alternate, or smoothly rotate in a helix, which results in a wide range of smectic phases.
Although they represent only two examples of the many LC phases discovered until now, nematic and smectic phases are the most widely studied.
Liquid Crystals and Light
As mentioned above, the ordering of molecules is high enough to confer LCs with quite captivating properties when compared to isotropic liquids. Because of the molecular order, all the directions in the liquid crystal are not equivalent, and this anisotropy is reflected in the electric, magnetic, and optical properties of the materials. Electric and magnetic anisotropy, along with the fluidity of the phase, result in the power to control the orientation of the LC by application of electric and magnetic fields. This, together with the optical anisotropy, gives us the perfect combination.
If the material is isotropic, the refractive index n is independent of the direction in which the light travels (propagates), and hence the polarization of light crossing the material is maintained. In contrast, with anisotropic materials such as liquid crystals all the directions are no longer equivalent and light coming into the material from different directions will "see" the molecules from different angles. For an arbitrary propagation direction, the electric field of a linearly polarized light wave can be divided into two components polarized along perpendicular directions. Each component "sees" a different refractive index; that is, one "travels" faster than the other and a phase shift is introduced between them. The difference between both indices ∆n (the birefringence), the sample thickness, and the light wavelength λ determines the magnitude of the phase shift, which modifies the polarization of the light wave that exists in the material.
Now imagine that we place a thin slice of isotropic material between crossed polarizers in the microscope. Because of the polarizer located between the light source and the sample, the light entering the sample is linearly polarized. As the polarization in this case is maintained, no light will be transmitted when arriving at the perpendicular polarizer. Now we will use a nematic LC slice instead. When passing through the LC, the polarization is changed and therefore, a component of the outgoing light wave can be transmitted through the polariser behind the sample. Indeed, the possibility of controlling the transmitted intensity by reorienting the material, and with it the relative light propagation direction, with small electric fields, is the foundation for the widespread use of liquid crystals in LCDs (Liquid Crystal Displays).
The microscopic image formed by the thin liquid crystal slice between crossed polarizers is commonly known as texture and is the fingerprint of the LC structure, and thus, as it was for Otto Lehmann, polarizing optical microscopy is the first tool employed for characterizing a new sample. Aside from providing important information, the liquid crystal textures are also beautiful and fascinating.
The Importance of Molecular Shape
The liquid crystal phases that appear depending on the temperature (in the process described above) consist of organic molecules, usually combining different phenyl groups directly linked or spaced by linking groups and flexible alkyl chains. Molecules that are narrower or wider in one direction than others have shown a strong ability to promote LC phases. Traditionally, LCs were limited to rod-like molecules and disc-like molecules. However, over the years, organic chemists have synthesized many other molecular shapes that show LC phases. This is the case with some polymers (provided they contain a LC unit), dimers (two units joined by an alkyl flexible chain), and bent-core molecules (often known as "bananas" because of their shape).
Each molecular shape has a strong impact on the way the molecules self-arrange and different nematic phases have been discovered among them over the years. While rod-like molecules show nematic and smectic phases as described above, disc-like materials show nematic and columnar phases. The addition of a chiral group into simple rod-like molecule gives rise to the chiral version of the nematic phase (N*) in which the preferred orientational direction gradually rotates to form a helix (see above image, top-right). "Bananas" also arrange themselves in nematic phases but show a strong tendency to form polar smectic phases. At the beginning of the 2010s, a new nematic phase, in which the preferred orientational direction twists and bends (NTB), was found in certain dimers (see above image, bottom-left). The discovery of any new nematic phase always generates a lot of interest within the field, both from the fundamental science and the technological point of view. Two nematic phases eventually became the Holy Grail of the liquid crystal community: the biaxial nematic phase and the polar nematic phase.
The Polar Nematic Phase
There is no fundamental reason why a nematic liquid crystal cannot be polar. For years, theoreticians predicted this would happen in a variety of systems, including rod-like and pear-like molecules, but it was never experimentally observed. Generally, it is more energetically favourable for rod-like molecules to orient in an antiparallel way, which prevents polar order. While ferroelectric and antiferroelectric order became "easily" accessible in smectic phases as early as the 1980s, nematic liquid crystals remained nonpolar until recently.
Several years ago, Richard Mandle, a chemist at Leeds University (UK) searching for materials with larger dipole moments for liquid crystal applications, synthesised a new organic molecule that clearly showed a phase transition between the N phase and what looked to be something almost identical to the N phase. When we heard of this development at the Jožef Stefan Institute, it immediately caught our attention, and we decided to investigate this new material that is comprised of slightly wedge-shape molecules (i.e. bulkier at one end than the other). Through a series of measurements, we concluded that in order to better fill the space, molecules have to splay in an alternating way leading to a modulated phase with alternating "stripes" where molecules point in the same direction (head-tail symmetry is broken and we have polar order) separated by regions where the head-tail inversion symmetry is recovered (see above image, bottom-right). This results in a modulated polar nematic phase that we called the splay nematic phase (NS).
We proved the polarity of the phase by using second harmonic generation (SHG) microscopy. SHG is a nonlinear optical process in which two photons of frequency ω that enter the material give rise to one photon of frequency 2 ω, i.e., two "red" photons combine to give rise to one "blue" photon. SHG can occur only in structures without inversion symmetry. A uniform normal nematic phase will only show a black image, while a polar phase will show an SHG texture defined by the polar structure. In fact, the splay nematic phase showed at the transition an SHG texture characterized by clear stripes 4-5 µm wide and delimited by dark stripes. Although at lower temperatures the texture becomes uniform, the SHG signal continues to increase, unambiguously indicating that this new nematic phase is polar.
This discovery has profound implications, both for fundamental science and for technological applications. On the one hand, plenty of work remains to be done before we determine which exact mechanisms and molecular interactions favour the transition to this phase and to the realization of truly ferroelectric liquids. On the other hand, nematic phases with polar order open the door for the faster manipulation of molecular orientation and the use of less power, features that are promising for rapid electro-optic switching energy efficient devices as well as for new LC photonic technologies such as switchable optical frequency converters.