I remember a warm summer evening when I was very young, still in kindergarten, maybe even younger. My mother rushed me to the shore of the sea to witness "something strange, something interesting". We went to an old stone bridge over a long and narrow bay. Throughout the evening, we watched the sea below the bridge rush into the bay for a couple of minutes, and then for another couple of minutes rush out of the bay – again and again. It looked like a river with the water running both up and downstream. This took place in Vrboska, my grandmother's village, a small and picturesque settlement on the northern coast of Hvar Island in the Adriatic Sea with old stone houses built along both sides of a bay, three old bridges and a new one crossing the body of water (Figure 1). It is an almost forgotten childhood memory. Little did I know then that what we were watching was probably a weak meteorological tsunami (meteotsunami), a phenomenon that I would come to investigate in detail for my masters and PhD theses, and as part of many other scientific projects, the most recent being SHExtreme, a European Research Council project that deals with meteotsunamis recorded on all the European coasts.
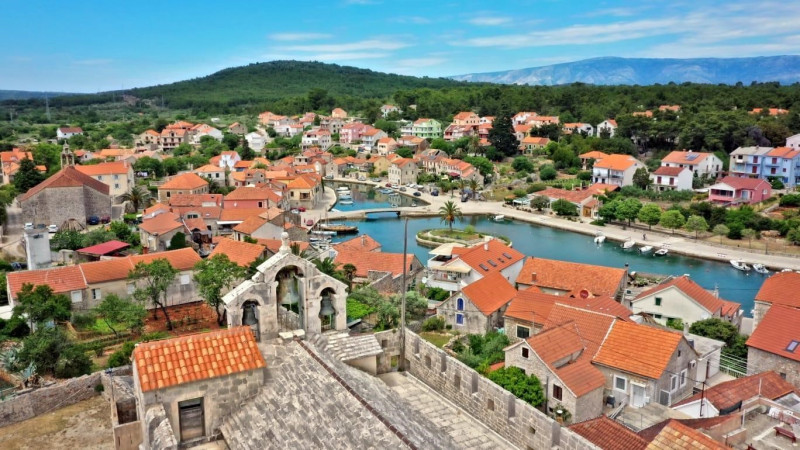
Figure 1: Photograph of Vrboska Bay, courtesy of Jure Erceg
Meteorological tsunamis are tsunami-like waves (Figure 2) of atmospheric origin. They have period, wave height, and energy content similar to what is observed in tsunamis of earthquake and landslide origin (Figure 2). Fortunately, meteorological tsunamis are not as destructive as the stronger earthquake or landslide tsunamis. Nonetheless, meteotsunamis have occasionally claimed human lives: specifically in the Great Lakes (Ewing et al. 1954), Algeria (Okal 2021), Japan (Hibiya and Kajiura 1980), and Korea (Okal 2021).
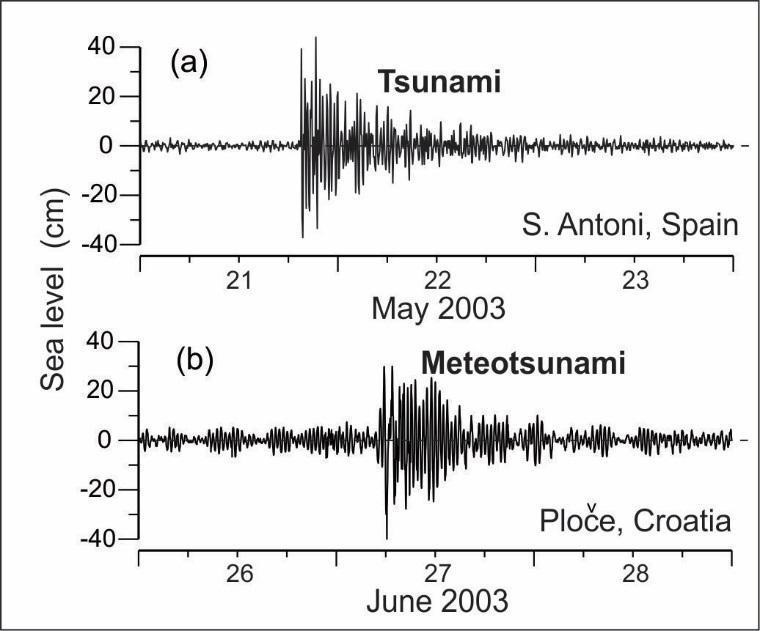
Figure 2: (a) Tsunami oscillations recorded at Sant Antoni (Ibiza Island, Spain) after the Algerian earthquake of 21 May 2003; and (b) the meteotsunami recorded at Ploče Harbour (Croatia) on 27 June 2003 (taken from Monserrat et al. 2006). Both series have been high-pass filtered.
The strongest meteotsunamis are generated through a sequence of steps summarized below and in Figure 3:
1) Generation and propagation of an atmospheric pressure disturbance – a small scale atmospheric pressure disturbance that is short but intense must exist in the atmosphere and propagate over the open sea. Such a disturbance is characterized by a relatively small change in air pressure (~2-5 hPa) that takes place over a relatively short period of time (~10-20 minutes) and on a relatively small spatial scale (~10-20 km). Despite the smallness of these factors, the resulting spatial and temporal rates of air pressure change can be quite large (Figure 2).
2) Proudman resonance – the resonant growth of long ocean waves that occurs when the speed of atmospheric pressure disturbances mentioned above matches the speed of the long waves, the latter being a function of sea depth. Depths from thirty to a hundred meters are the most favourable for the generation of meteotsunamis.
3) Shelf amplification – further amplification of long ocean waves that occurs as a result of the shoaling effect.
4) Harbour resonance – final amplification of long ocean waves in a bay that occurs as a result of the synchronization of periods of incoming long ocean waves and periods of the bay's standing waves.
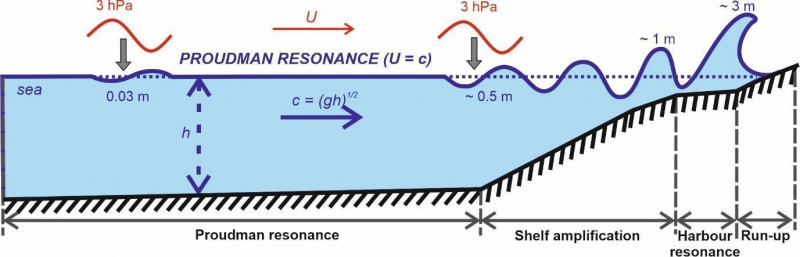
Figure 3: Illustration of meteotsunami generation processes. Atmospheric pressure disturbance propagating with a speed of U (represented by the red wave) generates long ocean waves that are amplified through the following mechanisms: (1) Proudman resonance, (2) shelf amplification, and (3) harbour resonance. The numbers in the figure above are for illustration only, the actual rates being dependent on the properties of the atmospheric pressure disturbance as well as the bathymetry and topography of the impacted area. Figure adapted from Šepić et al. 2016
Records of sea level and air pressure measured during a meteorological tsunami observed in Vela Luka (Korčula Island, the Adriatic Sea) are provided in Figure 4. It can be clearly seen that the intensification of air pressure oscillations at periods of ten to one-hundred and twenty minutes is followed by the intensification of sea level oscillations at the same periods. If there were no additional resonant effect, the recorded change of air pressure (1-2 hPa) would manifest in a change of sea level of one to two centimetres, while sea level oscillations of almost one hundred centimetres (from ~-60 cm to ~+40 cm) were recorded.
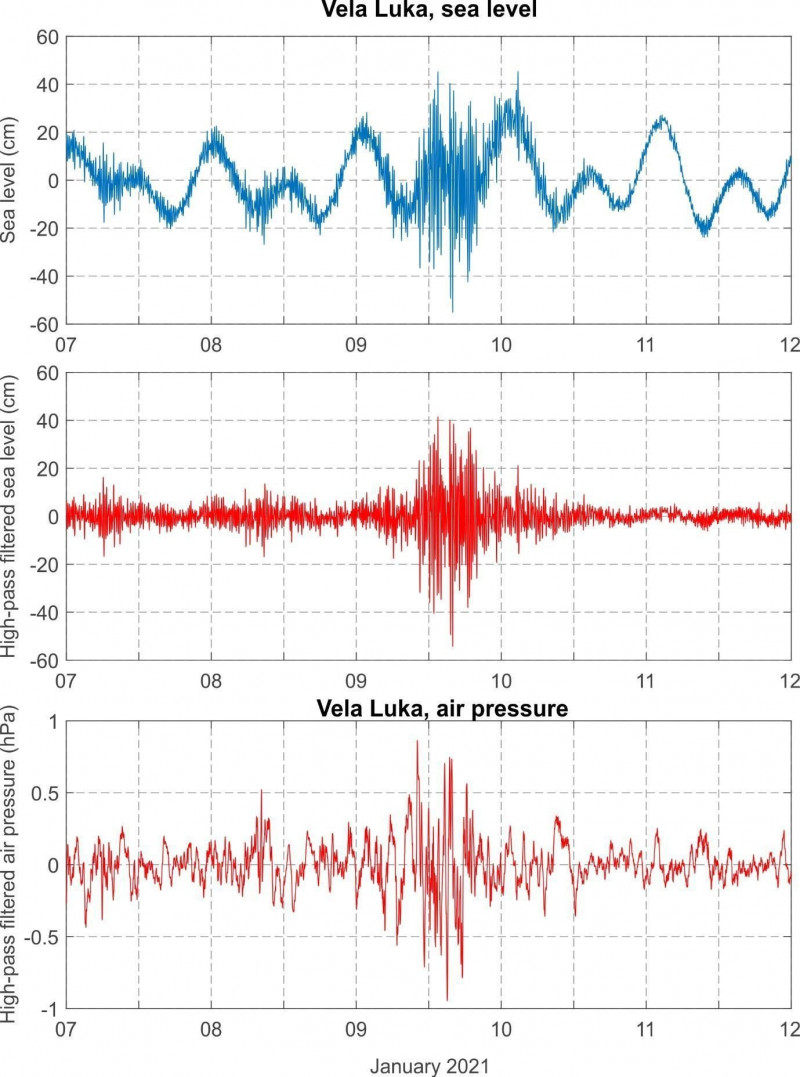
Figure 4: Original (top chart) and high-pass (middle chart) filtered sea-level time series and high-pass filtered air pressure time series (bottom chart) measured at Vela Luka during the 9 January 2021 meteotsunami event. Figure taken from Šepić et al. 2022
The specific conditions needed for the generation of a strong meteotsunami do not often occur. For this reason, strong meteotsunamis are not a common phenomenon in most of the world. Nonetheless, there are certain locations called meteotsunami hotspots where meteotsunamis occur more frequently and are stronger than average. One meteotsunami hotspot is the Mediterranean Sea, and in particular the Adriatic Sea (Figure 4). Many bays on the eastern coast of the Adriatic Sea are hit by weak to moderate meteotsunamis once every few years, with some bays experiencing truly destructive meteotsunamis once every few decades. The Adriatic meteotsunami catalogue lists thirty-six meteorological tsunamis that occurred on the eastern Adriatic coast between 1931 and 2021. Most of the events were observed in Vela Luka (eighteen events), followed by Stari Grad on Hvar Island (eleven events), and Vrboska (seven events).
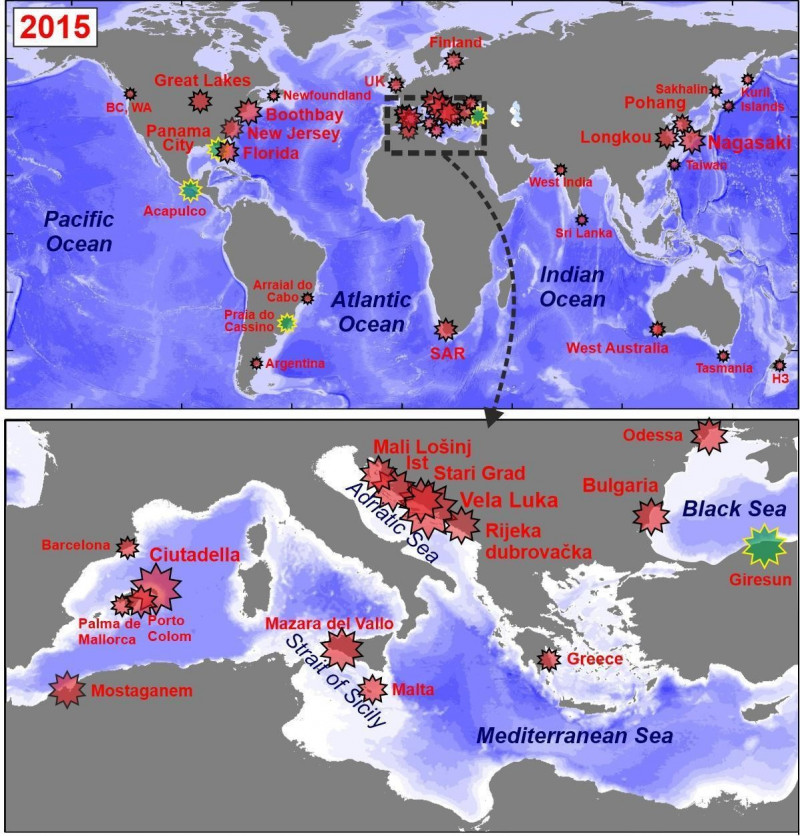
Figure 5: Known locations of meteorological tsunamis in 2015. The size of the star is proportional to the height of the event. Green stars mark events that were only suspected to be meteotsunamis. Figure adapted from Vilibić et al. 2016
The strongest and most devastating of known Adriatic meteotsunamis occurred on 21 June 1978 in Vela Luka (Figure 6). During the early morning hours, the sea level in Vela Luka suddenly began to rise. Within ten minutes the ground floors of houses located at the top of the bay were completely flooded. After the sea reached its highest level, it dramatically retreated, completely emptying the narrow inner harbour in ten minutes, leaving all the boats stranded at the now dry sea bottom (Figure 6). Then the sea rushed back in. Throughout the entire morning, the sea went in and out of the harbour in a repetitive dance of destruction (Vučetić et al. 2009). Two years later, in 1980 Professor Mirko Orlić hypothesized that the event was generated by propagating atmospheric pressure disturbances. The disturbances were so small that they were hardly detectable on the low-resolution paper and ink air pressure charts of that time. Yet all later analyses, including numerical modelling, confirmed Orlić's hypothesis. The Great Vela Luka Flood of 1978 event remains the strongest known meteotsunami.
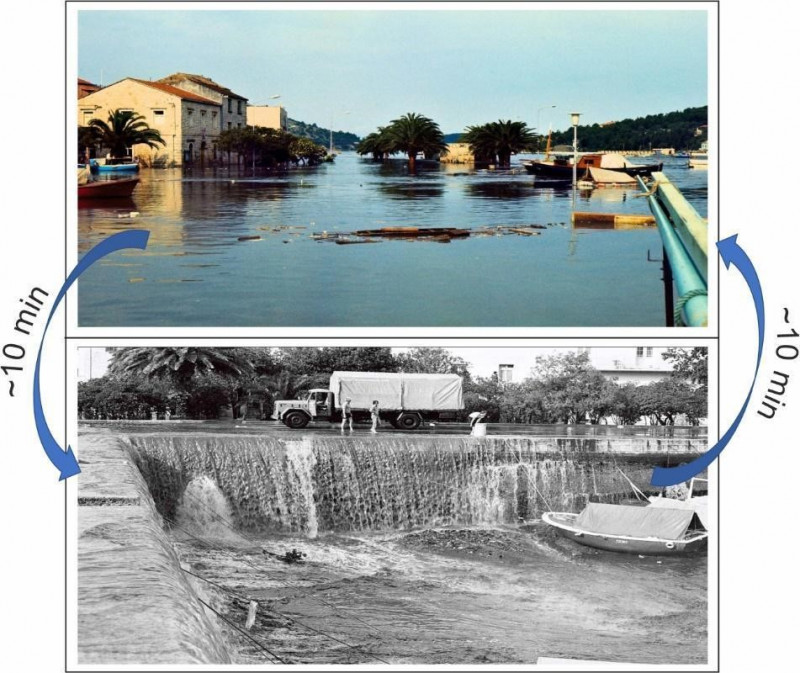
Figure 6: Photographs of Vela Luka taken during the morning hours of 21 June 1978: the flooded Vela Luka Bay in the first image, the drained Vela Luka Bay in the second image. Photos: the municipal photo archive of Vela Luka (HR-DADU-SCKL-692, Vladimir Stanišić)
There are many reasons for the abundance of meteorological tsunamis in the Adriatic Sea: frequent atmospheric pressure disturbances (step 1) capable of triggering meteotsunamis are generated over the Apennine and Alpine mountains ranged during the warmer part of the year. The direction and speed of these disturbances are occasionally sufficient to trigger the Proudman resonance (step 2), the result being the generation of open sea tsunami-like waves accumulating towards the eastern Adriatic coast. Upon reaching the eastern Adriatic coast, the tsunami-like waves first strengthen due to the shoaling effect (step 3), and then further intensify in bays and harbours of specific shape (step 4). A detailed study of navigational charts reveals that most of the meteotsunami-prone locations in the Adriatic Sea (Vrboska, Bakar, Vela Luka, Stari Grad, Mali Lošinj) are long and narrow bays. Although this shape effectively protects the bays (and the numerous towns and harbours in such bays) from wind waves, it also tends to strengthen rare tsunami-like ocean waves, which occasionally grow into meteotsunamis of destructive dimensions.
A significant effort has been invested in the development of an Adriatic meteotsunami research and warning network. Most of the work has been done at the Institute of Oceanography and Fisheries in Split. Thus far, seven tide gauge stations measuring one-minute temporal steps and nine microbarograph stations measuring air pressure with one-second temporal resolutions have been installed at meteotsunami-prone locations. These installations allow for the real-time assessment of danger at these locations as they monitor downstream measurements of the atmospheric/ocean flow. By observing what is happening in Ancona, Italy and other remote stations, we are able to anticipate what will happen in Vela Luka one to two hours later.
In addition, an assessment of the synoptic conditions related to the Adriatic meteotsunamis has been performed (Vilibić and Šepić 2009). It shows that Adriatic meteotsunamis commonly occur during the summer months when the following conditions are met:
1) a weak surface pressure is located over the Adriatic Sea or to the west of it;
2) an inflow of warm and dry air of African origin over the Adriatic Sea is detected in the lower troposphere (below 5,000 m);
3) a strong southwesterly jet stream (wind speeds above 20 m/s) is blowing over the Adriatic with a height of ~5,000 m;
4) this jet stream is embedded in a dynamically unstable atmospheric layer.
The conditions favour both the generation and propagation of atmospheric gravity waves that manifest at the surface as meteotsunamigenic air pressure disturbances. Finally, an atmospheric ocean model aimed at real time forecast of meteotsunamis was run for a period of time producing a statistical estimate of the probability of meteotsunami occurrence at specific eastern Adriatic locations (Denamiel et al. 2019).
Despite all of these efforts, the forecast of meteorological tsunamis remains a challenge, the main problem being the intrinsic irreproducibility and untraceability of exact meteotsunamigenic atmospheric pressure disturbances. This is perhaps best illustrated in our study in which we used measured air pressure data to force a high-resolution ocean model with the goal of reproducing a series of meteorological tsunamis that struck the Adriatic Sea in June 2014. In one experiment, we used air pressure data measured in Vrboska, and in another air pressure data measured some twenty-five kilometres from Vela Luka. Depending on the data used, the final height of the modelled meteotsunamis differed up to one hundred percent (Figure 7). This illustrates that the forecasting of meteotsunamis remains a challenge that we will be working on for many years to come.
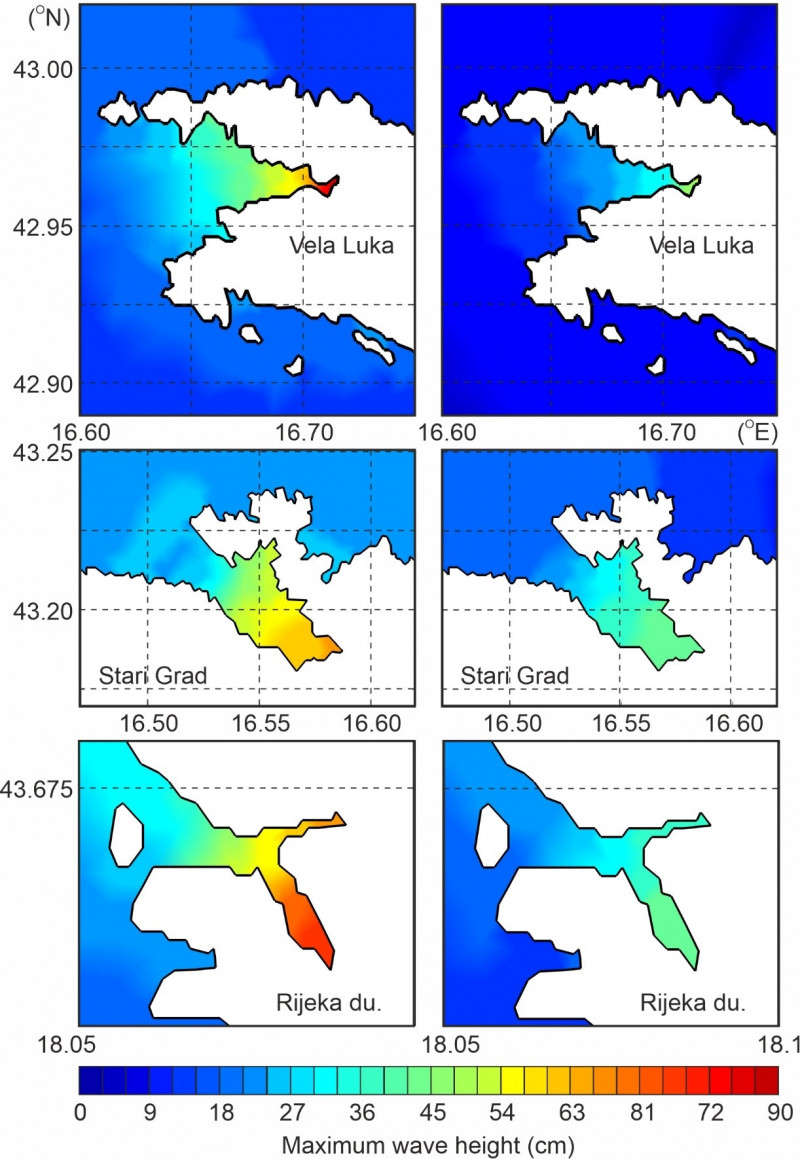
Figure 7: Left column: maximum wave heights modelled at Vela Luka, Stari Grad, and Rijeka dubrovačka bays during an experiment using air pressure time series measured at Vela Luka set to propagate a speed of 40 m/s and a direction of 270o. Right column: same as the left column, but with an air pressure time series measured at 25-km distance from Vrboska (Šepić et al. 2016).
But finally, if you find yourself in the sea, on the beach or at the coast, and you notice the sea suddenly approaching the coast, looking like a wall of water, or retreating from the coast, and uncovering the sea bottom, move to higher ground as soon as possible.